The Wonders of Electromagnetism
Discover the Workings of Highly Efficient Nuclear Batteries Used On Earth and In Space
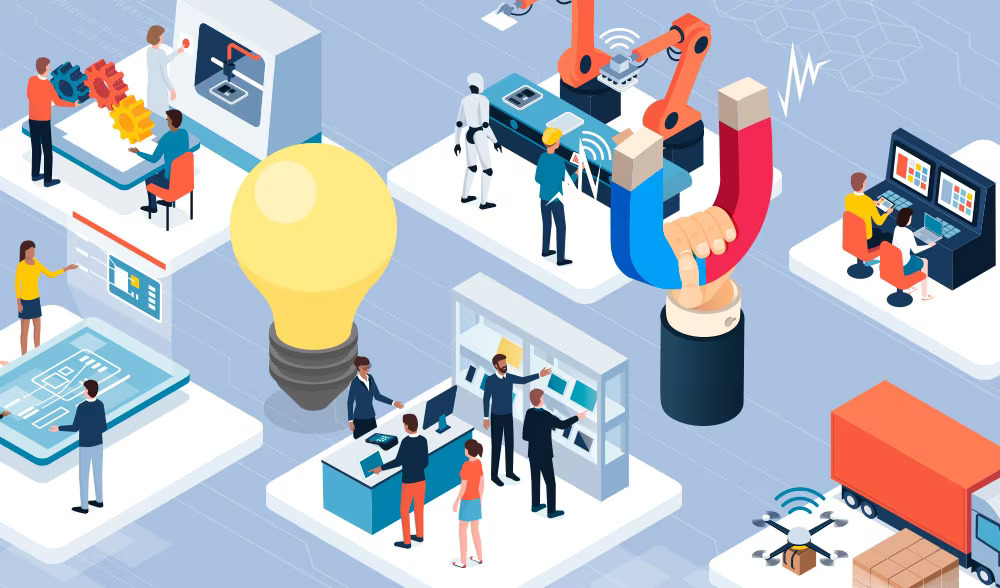
Planetary probes making lengthy journeys through deep space over years and decades cannot be reliably powered by conventional chemical batteries, which have limited lifespans, nor by solar cells. Nuclear batteries, which combine radioisotopes with thermocouples, provide the solution. Nuclear batteries—also known as atomic batteries, radioisotope batteries, or radioisotope thermoelectric generators (RTGs)—convert the heat generated by the decay of radioactive isotopes into electricity, offering the advantage of supplying steady power over extended periods. Thermocouples leverage the Seebeck effect, discovered over 200 years ago, and are undergoing continued research and development toward practical applications in the automotive and medical fields.
- Nuclear batteries use radioisotopes as a heat source
- Diamond batteries deliver longevity and stable, low-power output
- The thermocouple’s contribution to the discovery of Ohm’s Law
- Electronic cooling and heating using the Peltier effect
- Using thermoelectric generators to extract electric power from car exhaust heat
Nuclear batteries use radioisotopes as a heat source
Launched by NASA in 1977, the Voyager spacecraft embarked on a mission to explore Jupiter, Saturn, Uranus, and Neptune, capturing photographs along the way before venturing beyond the boundaries of our solar system, all while transmitting signals back to Earth.
Sending signals requires electrical energy. However, as a spacecraft moves farther away from the sun, solar energy diminishes, necessitating massive solar panels if it is to rely only on solar power. This is why planetary probes traveling beyond Jupiter are equipped with nuclear batteries. Nuclear batteries feature an exceptionally long lifespan: even after 45 years, those on Voyager continue to operate, enabling the spacecraft to transmit scientific data back to Earth.
In recent years, nuclear batteries have also been powering rovers exploring the surfaces of Mars and the Moon, which are closer to Earth than Jupiter. Unlike spacecraft continuously traveling through space, planets and moons experience day and night cycles as well as varying weather conditions. Consequently, there are periods during which solar power alone cannot provide sufficient electricity. With the lunar day-night cycle lasting approximately 28 days, relying solely on solar power limits operational periods to a maximum of 14 days. China’s unmanned rover, Yutu, deployed by the Chang'e 3 lunar probe launched in 2013, was equipped with nuclear batteries and set a lunar surface operational record of 972 days.
Initially, NASA used solar power for its Mars rovers. But the planet’s thin atmosphere and swirling dust on the surface risked contaminating the panels and preventing them from generating power. For this reason, the Mars rovers Curiosity, which arrived on Mars in 2012, and Perseverance, which landed in 2021, are powered by nuclear batteries. Similarly, NASA’s New Horizons, launched in January 2006 as the first spacecraft to explore Pluto, also utilizes a nuclear battery. Referred to as a radioisotope thermoelectric generator (RTG), it is a black, cylindrical structure protruding from the spacecraft’s fuselage, resembling a chimney.
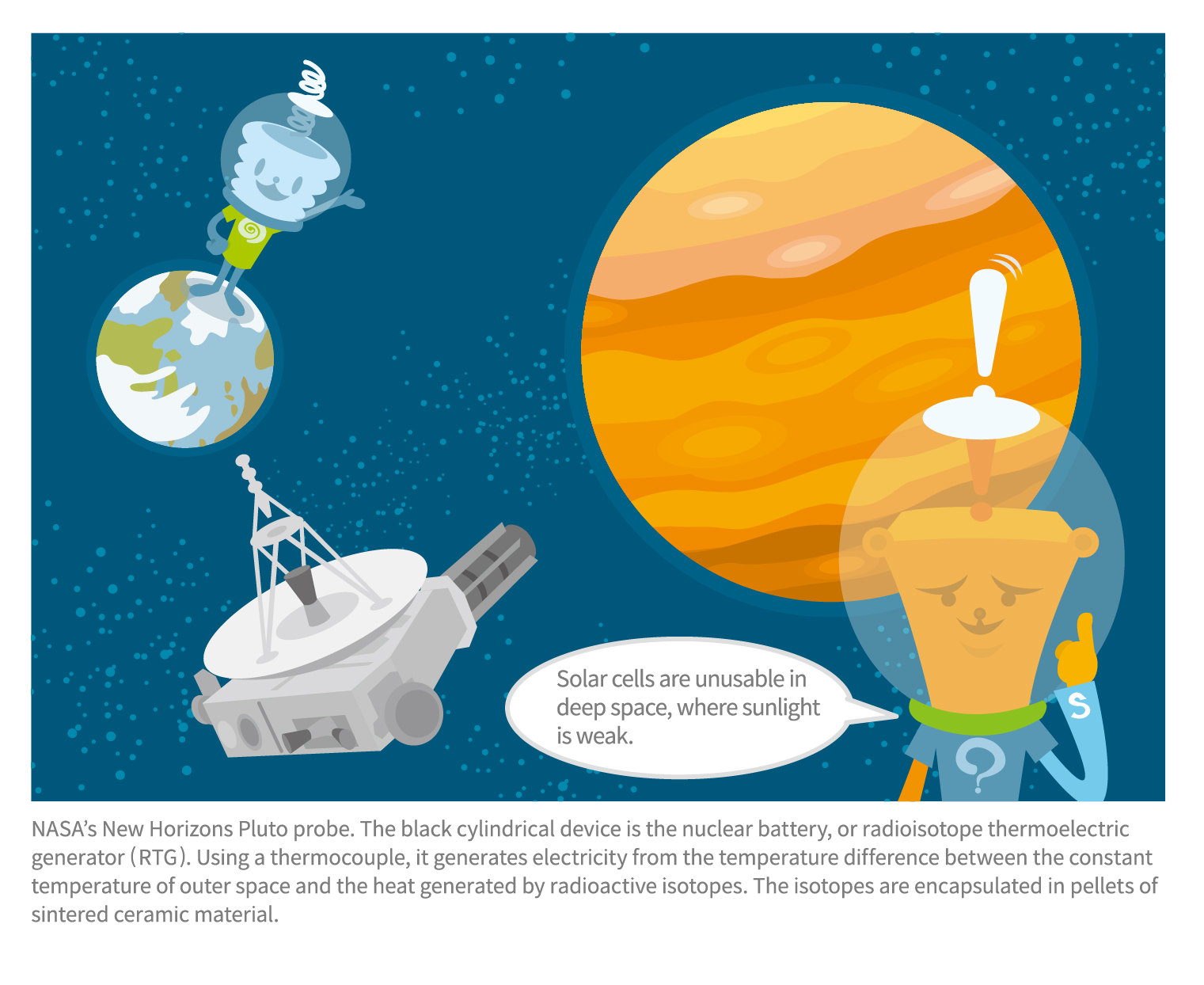
Nuclear batteries are electric power generators based on thermocouples. A thermocouple consists of a loop formed by joining different types of metals. When one junction is heated, it generates an electromotive force, creating an electric current. This phenomenon is known as the Seebeck effect, named after its discoverer, German physicist Thomas Seebeck.
A nuclear battery combines thermocouples and radioactive isotopes. When radioisotopes undergo spontaneous decay, they emit radiation, which generates heat upon being absorbed by a substance. This heat is then converted into electricity through thermocouples, which is why they are also referred to as radioisotope batteries.
Nuclear batteries use isotopes such as plutonium-238 (half-life: 87.7 years). Since a nuclear battery’s principle and structure are fairly simple, it is technically feasible to create extremely compact versions. In fact, some were even developed for use in pacemakers in Europe and the United States in the past. However, the main drawback is concerns regarding radiation exposure, making their use in consumer devices challenging. Additionally, if a spacecraft equipped with a nuclear battery were to fall on Earth, there is a risk of widespread environmental contamination from the dispersal of radioactive material. Therefore, nuclear batteries are limited to specific applications and require safety measures such as encapsulating the radioactive material in pellets and enclosing the device in rugged containers.
Other potential applications of nuclear batteries include sensors and monitoring equipment used in environments where maintenance is difficult, as well as research instruments deployed in harsh environments like the deep sea. Notably, Japanese space exploration probes currently do not utilize nuclear batteries.
Diamond batteries deliver longevity and stable, low-power output
Another technology attracting attention for generating electricity from isotope decay is diamond batteries. In 2016, a research team at the University of Bristol in the United Kingdom developed a prototype diamond battery using synthetic diamonds containing carbon-14, marking a significant step toward practical application. Research and development continue today to improve output efficiency, enhance safety, and reduce costs.
The basis of diamond batteries lies in the electric energy generated when electrons produced from the decay of isotopes pass through diamond. Carbon-14 has a half-life of approximately 5,730 years—much longer than plutonium-238 (87.7 years) used in nuclear batteries—making it a long-lasting power source capable of supplying electricity for millennia. Additionally, carbon-14 is a relatively safe radioactive material abundantly found in nature. Diamond batteries are inherently very safe because they involve no chemical reactions, minimizing the risks of explosion or leakage, and also because diamonds naturally shield radiation.
Diamond batteries, with lower output compared to nuclear batteries, are expected to find utility in applications such as implantable medical devices. Nuclear batteries, on the other hand, are better suited for applications with higher energy requirements, such as space exploration and remote monitoring equipment.
The thermocouple’s contribution to the discovery of Ohm’s Law
The Seebeck effect, the theory behind the thermocouple, was discovered in 1821, the year after Hans Ørsted’s experiment demonstrating the magnetic effect of electric currents. At the time, experiments in electrical phenomena commonly used voltaic cells constructed of zinc and copper electrodes immersed in dilute sulfuric acid. Seebeck wondered if it could be possible to generate electricity by simply bringing two different metals into contact without immersing them in a liquid. To test this hypothesis, he constructed an experimental apparatus using a coil of copper wire, bismuth (or antimony), and a magnetic needle (see Figure A).
Merely bringing copper and bismuth into contact does not produce any electric current. Fortunately for Seebeck, when he coincidentally pressed down on the copper and bismuth with his fingers, he noticed a faint movement of the magnetic needle. Yet, when he tried pressing them together using a glass rod instead of his fingers, the needle remained motionless. Guessing that the heat from his fingers might be a factor, Seebeck devised a famous experimental apparatus in which copper and bismuth plates were soldered together to form a loop (see Figure B). When one side of the junction was heated using an alcohol burner, a current flowed through the loop, causing the magnetic needle to move. Seebeck also discovered that cooling one side of the junction generated a current flowing in the opposite direction.
Characteristically, in a thermocouple, the combination of the metals used and their temperature difference precisely determines the electromotive force generated. Therefore, an extremely stable voltage can be obtained if the temperature difference between the two junctions is kept constant. This finding on thermocouples led to the discovery of Ohm’s Law, one of the most fundamental laws of electricity.
Georg Ohm conducted experiments by connecting copper wires of the same thickness but of varying lengths (as a means to adjust resistance) to investigate how the intensity of electric current is affected, measured by the deflection of a magnetic needle. However, he could not obtain sufficient data using voltaic cells because their voltage was not stable enough. Instead, he turned to Seebeck’s thermocouple. He kept the temperature difference constant by setting one end of the thermocouple in boiling water (100°C) and the other end in ice water (0°C), which yielded a remarkably stable voltage (see Figure C). The experimental data from this apparatus was what led to the 1826 discovery of Ohm’s Law: Current = Voltage / Resistance.
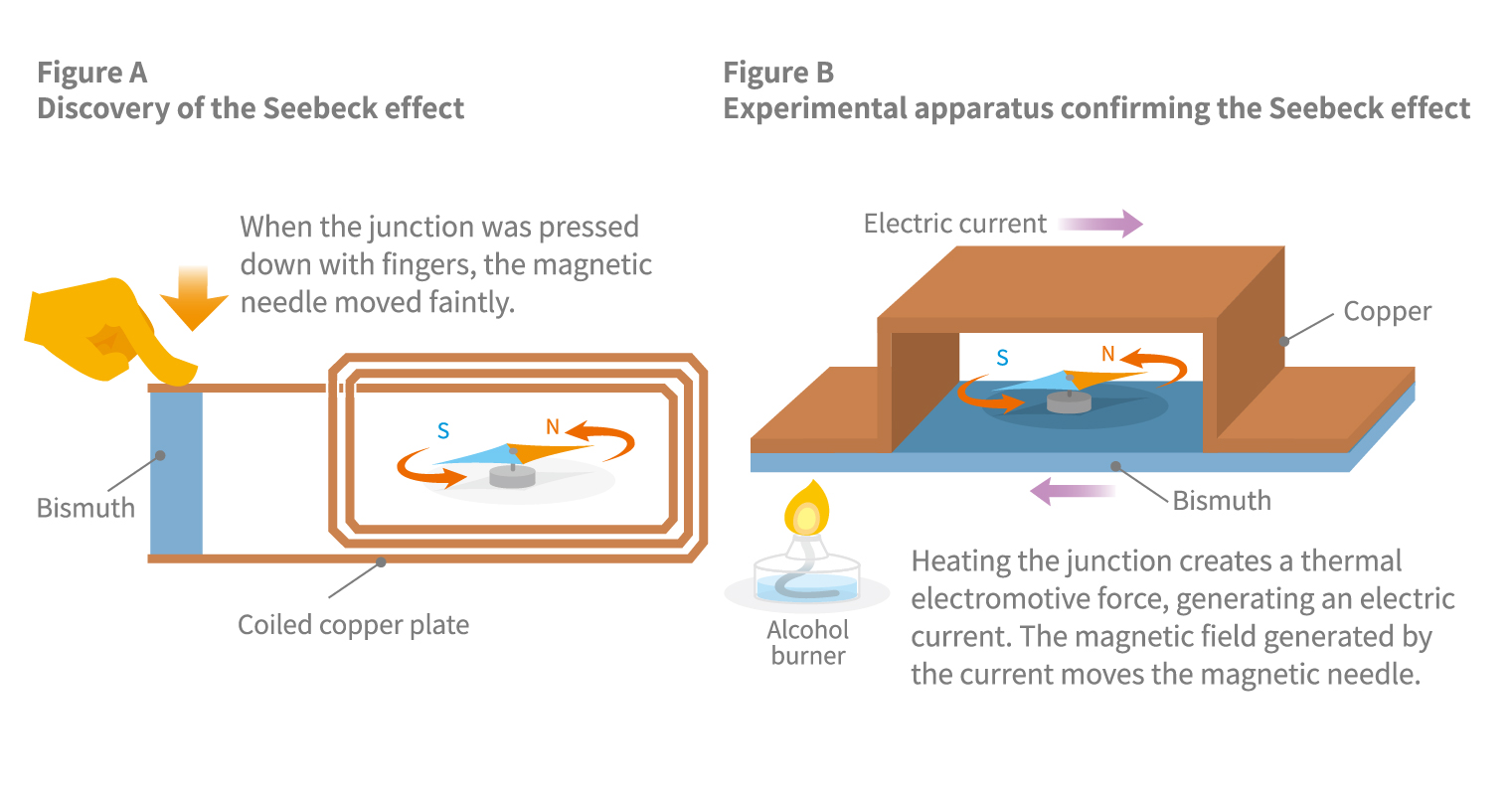
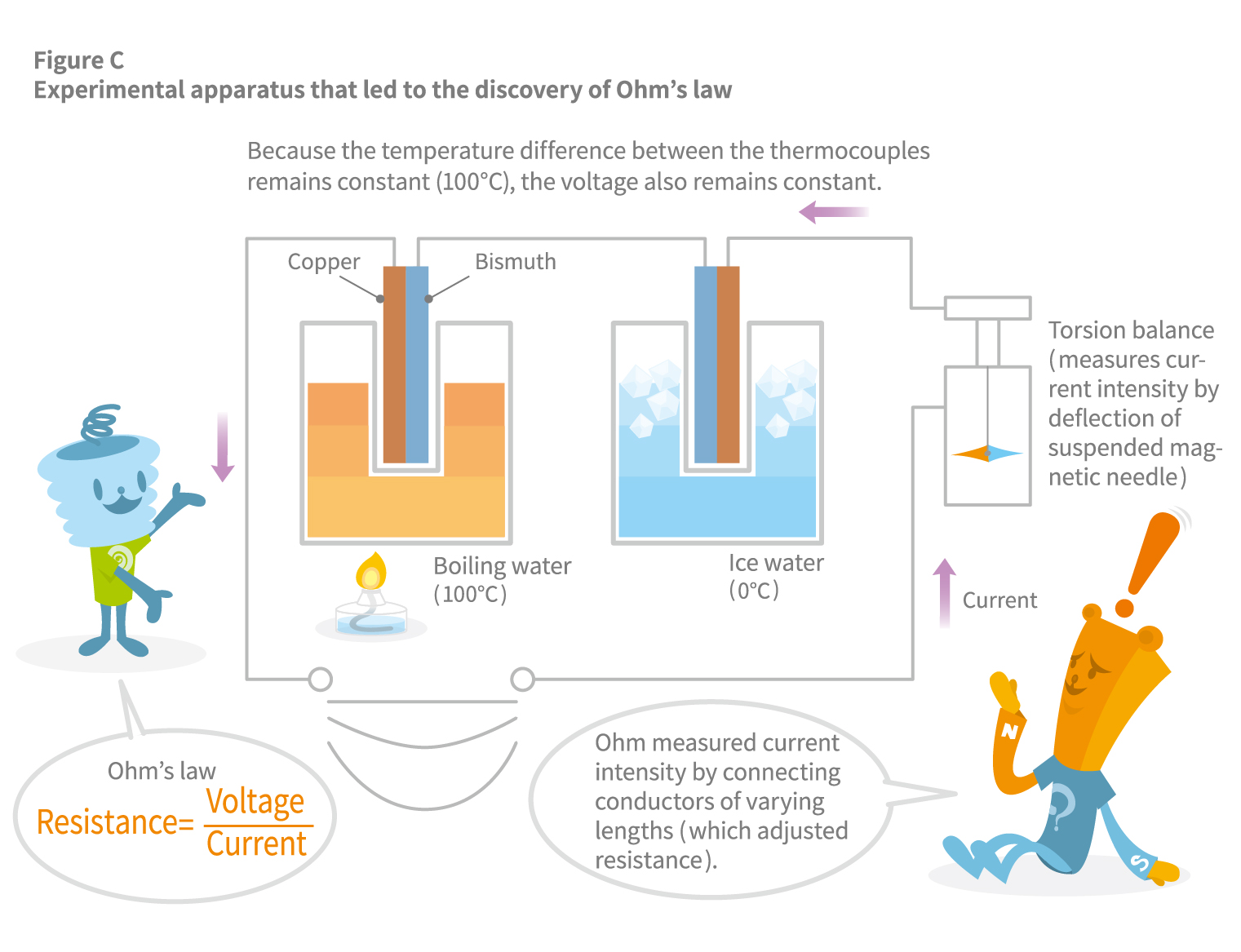
Electronic cooling and heating using the Peltier effect
The French scientist Peltier conceived that if a temperature difference between two different metals could generate an electric current, then conversely, an electric current may create a temperature difference between the two metals. In 1834, Peltier fabricated a rod on which antimony and bismuth were soldered and confirmed that passing a current from the antimony side caused it to heat up, while reversing the direction resulted in cooling. This phenomenon is known as the Peltier effect (see Figure D).
The Seebeck and Peltier effects are part of a physical phenomenon collectively known as the thermoelectric effect. The mutual interaction between heat and electric current occurs because electrons serve as carriers of both electric current and of heat. Accordingly, the thermoelectric effect can be produced not only by two metals but also by two types of semiconductors.
For example, when N-type and P-type semiconductors are arranged alternately and sandwiched between metals, one side of the metal becomes a cooling surface while the other becomes a heating surface (see Figure E). N-type semiconductors are characterized by an excess of electrons unbound to atoms and can freely move around, while P-type semiconductors have a deficiency of free electrons. When these two semiconductors are joined and a current is passed through them, a thermodynamic flow occurs. However, due to differences in how heat diffuses between the N-type and P-type semiconductors, cooling and heating effects are produced.
By harnessing this cooling effect, it is possible to create electric refrigerators that require no refrigerants. They are silent because there are no moving components like motors or compressors. Still, their power is limited, so they are typically only used as small refrigerators in hotel rooms or beside hospital beds. The article The History of Refrigerators and the Evolution of Refrigeration Technology broadly discusses the mechanism and history of refrigerators.
The Peltier effect is also utilized for cooling CPUs and GPUs in personal computers due to the small size and minimal vibration and noise. By bringing the cooling surface into contact with a CPU or GPU, heat can be dissipated directly, preventing overheating of the chips. Thermoelectric cooling is also found in polymerase chain reaction (PCR) testing equipment, widely deployed during the COVID-19 pandemic. Because PCR testing involves DNA replication processes that require cycling through numerous temperature settings, testing equipment makes use of Peltier elements to alter temperatures rapidly.
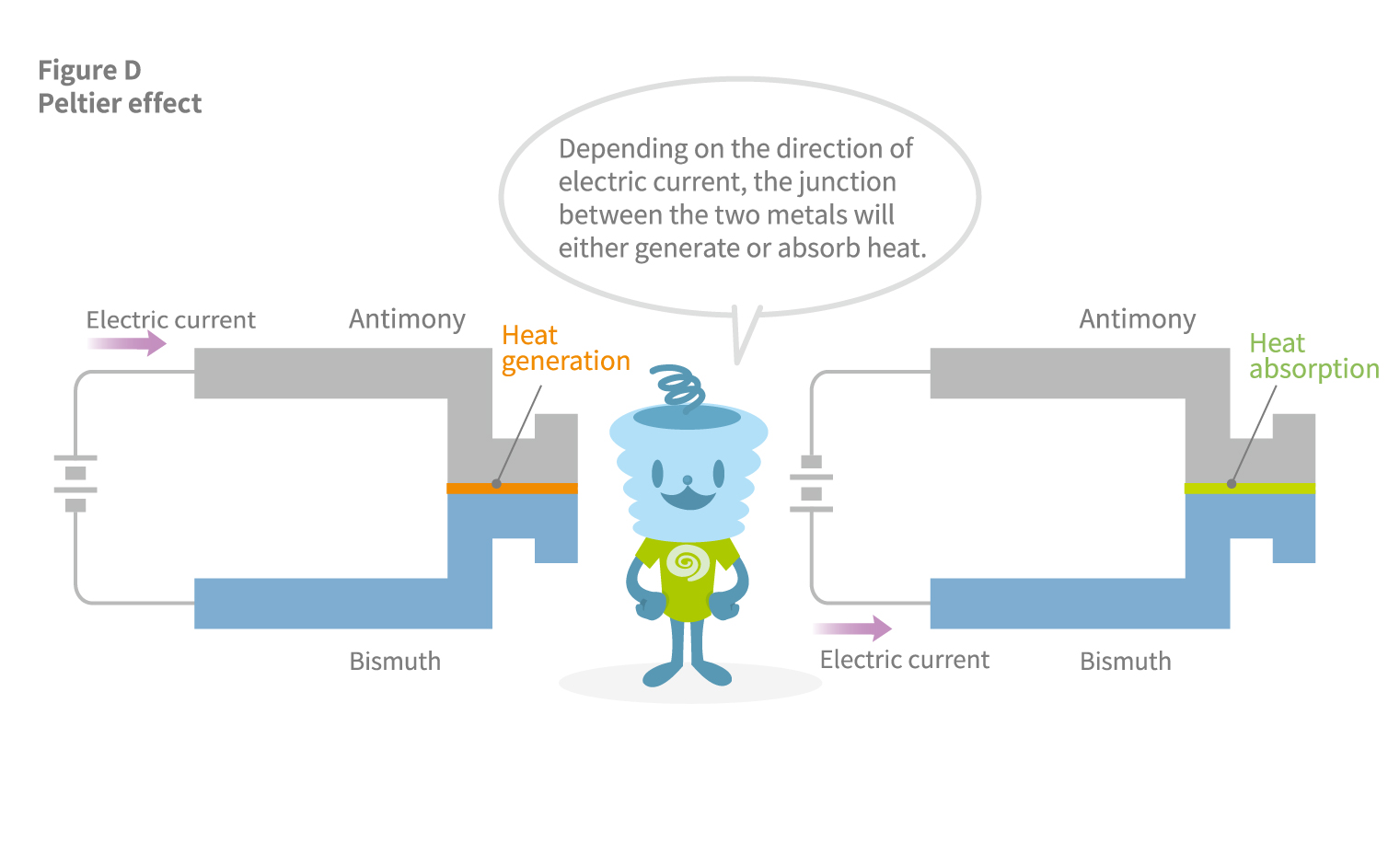
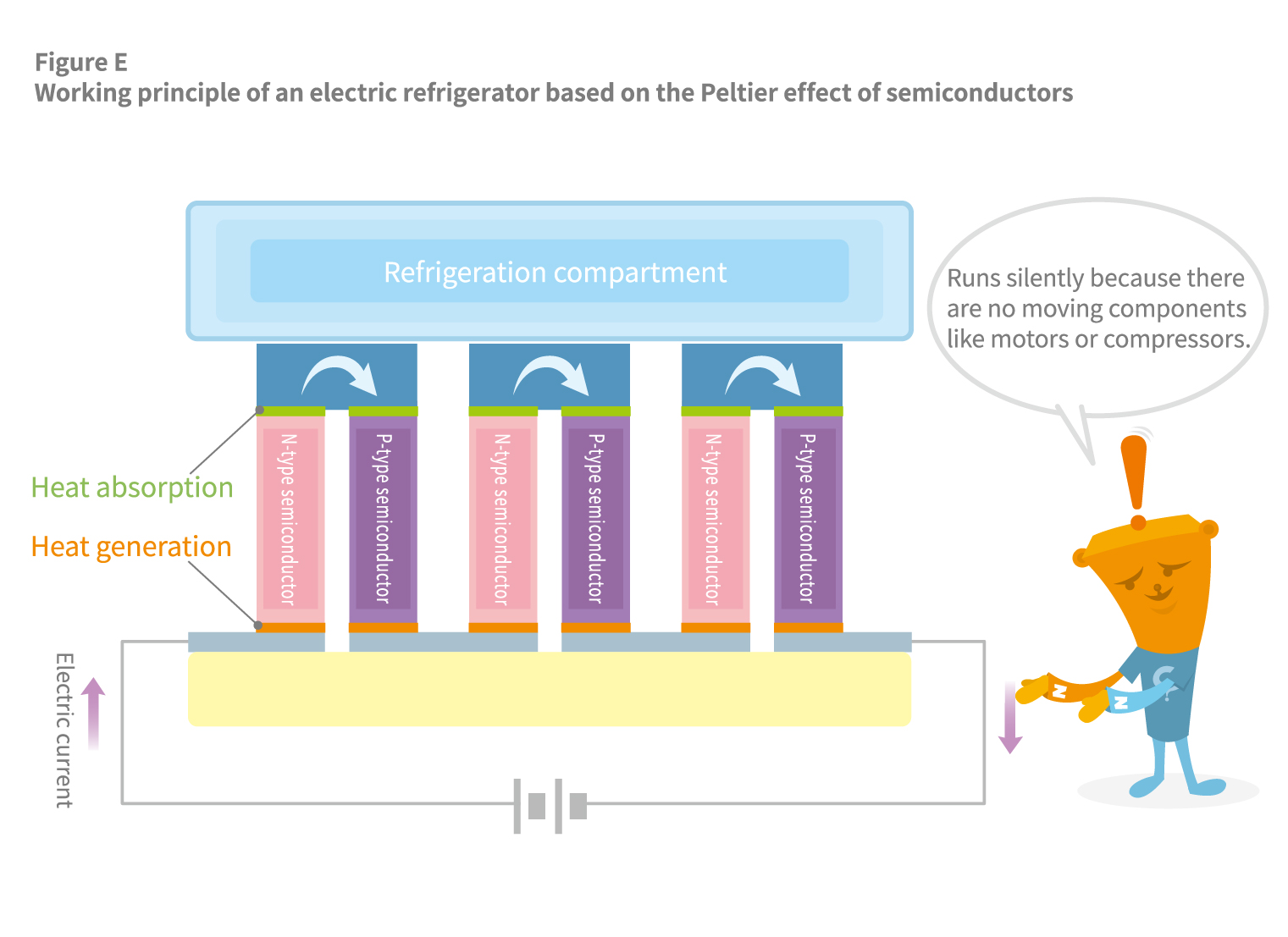
Using thermoelectric generators to extract electric power from car exhaust heat
An application of semiconductors’ thermoelectric effect can be found in automobiles today. Discarding the high-temperature exhaust gases from the engine is tantamount to wasting energy. By employing thermoelectric conversion elements formed from P-type and N-type semiconductors, electricity can be generated directly from waste heat, partially supplying the power required by onboard electrical equipment. This innovation in energy-saving technologies in automobiles and other industries continues to advance and enable new solutions with each passing year.
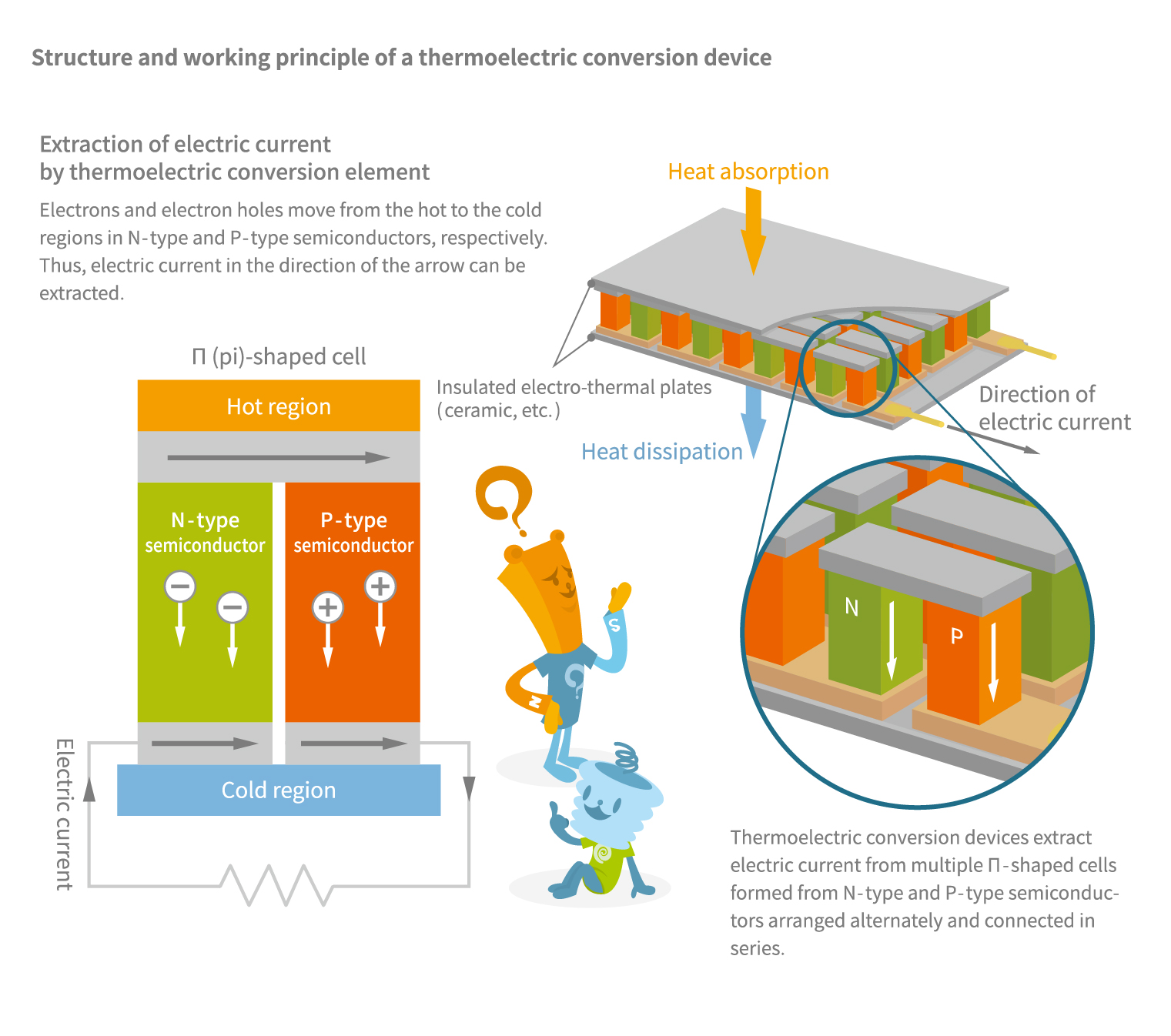
TDK is a comprehensive electronic components manufacturer leading the world in magnetic technology